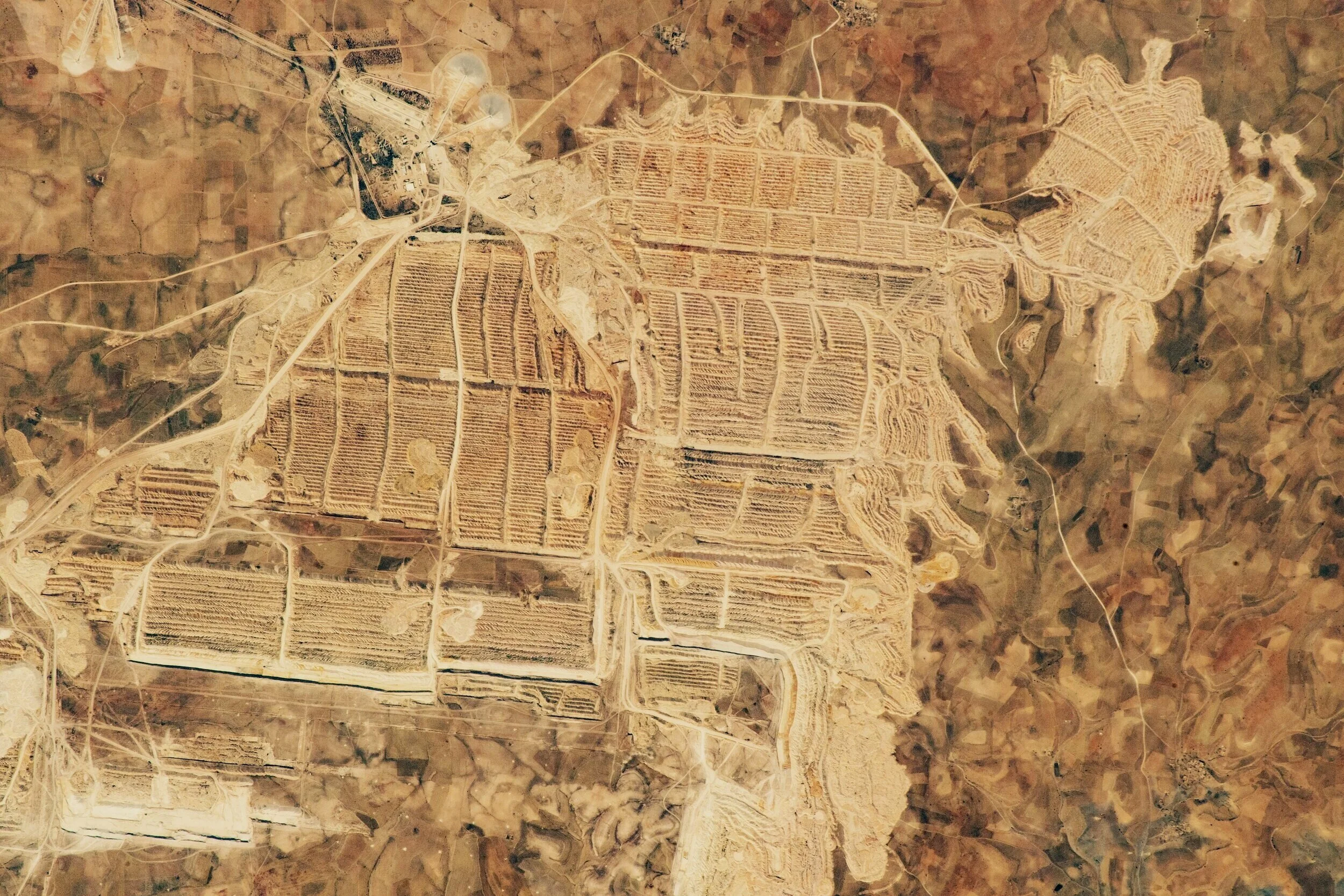
Chapter 2 summary
Phosphate rock: resources, reserves and uses
Chapter authors: Will J. Brownlie, Mark A. Sutton, Marissa A. de Boer, Lino Camprubí, Helen A. Hamilton, Kate V. Heal, Tibisay Morgandi, Tina-Simone Neset, Bryan M. Spears • 10min read
Chapter highlights
Five countries hold 85% of the planet’s phosphate rock reserves. High dependency on imported phosphate rock and/or mineral phosphorus fertiliser can contribute to national food system vulnerability. Geological depletion of phosphate rock is not an immediate threat, however geopolitical, institutional, economic, and managerial factors may impact phosphorus access. Improving the efficient use of phosphorus in agriculture and shifting reliance away from mined phosphorus sources by increasing phosphorus recycling may offer the greatest protection against potential phosphorus supply risks.
Introduction
Why do we need to improve the management of our phosphate rock reserves?
For millions of years, the total amount of ‘mobile’ phosphorus supporting the world's ecosystems remained largely unchanged. This ‘mobile’ phosphorus flowed between the various compartments of soil, plant, animal, wastes, waters, and sediments. Around 8000 years ago when farmers discovered that applying animal manures to croplands improved their harvests, humans started manipulating this system. In the 19th century, in addition to manures, phosphorus fertilisation of crops was commonly achieved by applying bone meal from slaughtered animals to soils. However, as food demands increased, the low solubility of bone meal was not sufficient to provide an adequate supply of phosphorus to crops. By 1840, the agricultural scientist Justus von Liebig had discovered that acid-treated bone meal released phosphorus more readily to soils, increasing phosphorus uptake by crops. In the same year, Liebig successfully applied the same acid treatment to phosphate rock. In the years that followed, John Bennet Lawes developed this process at an industrial scale and renamed the product ‘superphosphate fertiliser’; this was to be the first phosphorus fertiliser commercially produced worldwide. Since that time, with rapidly increasing production since World War II, phosphate rock has been the main source of phosphorus used by society, predominantly to provide fertilisers.
Over the past century, anthropogenic use of phosphorus, predominantly in agriculture, has increased by a factor of ~18. Globally, around 85% of phosphates produced for market are processed to make mineral phosphorus fertilisers, and 10% are used to make animal feed supplements. The remaining 5% is used across a range of chemical industries, from flame retardants to food additives. Demand for phosphate rock to supply fertiliser demands has increased rapidly since the 1950s. Over the past century, anthropogenic use of P, predominantly in agriculture, has increased by a factor of ~18 (from ~2 Mt in 1910 to a peak use of 36 Mt in 2016). This is primarily due to the increasing agricultural production that is required to sustain a growing human population with an increasing demand for animal products, non-food products and biofuel production.
In 2020, 30 Mt of phosphorus was mined from global phosphate rock reserves estimated to contain over 9000 Mt of phosphorus. Phosphate rock ‘reserves’ are defined as phosphate rock deposits from which phosphate rock can be economically produced. Based on rates of phosphate rock production for 2020, the ‘lifetime’ of existing reserves is 318 years. This contrasts with phosphate rock ‘resources’, which are defined as phosphate rock of any grade, including deposits that cannot be currently mined without significant economic and/or environmental cost. Global phosphate rock resources are estimated to contain some 40,000 Mt of phosphorus. As reserves are depleted and technological innovation occurs, some of these resources would be expected to be converted into minable reserves.
There is, therefore, no risk of exhausting global phosphorus supplies within the next 100-300 years. However, if mining production continues at the current rate, domestic supplies in the three countries with the largest populations - China, India, and the USA - will be depleted within 40 years. Similarly, at current mining rates three of the four countries that produce the highest quantities of phosphate rock globally (i.e. China, the USA and Russia) will have exhausted their phosphate rock reserves within the next 46 years.
While some of these time horizons may appear long, it is evident that the current linear economy of phosphate rock mining followed by the waste of lost phosphorus resources is ultimately unsustainable. Poor phosphorus management has resulted in widespread phosphorus losses to waterbodies, causing significant damage to rivers, lakes, and coastal waters. Reliance on mined sources of phosphorus will continue to mobilise phosphorus that would otherwise be ‘locked away’, into a global mineral cycle where elevated phosphorus flows are having major impacts on freshwater and marine ecosystems around the world. The human-driven release of phosphorus has been estimated to exceed the “planetary boundary” for freshwater eutrophication (i.e., beyond levels deemed safe to avoid abrupt, irreversible environmental change) by a factor of three. At the same time, current global reliance on mineral phosphorus for fertilisers to produce food means access to mineral phosphorus remains a critical requirement for food security at present. Actions to reduce the fraction of available phosphorus resources that are wasted by losses to the wider environment are necessary to address this dilemma, thereby moving towards a more circular economy for phosphorus, where recovery and reuse of phosphorus are central
In the following section, we discuss the challenges and solutions for managing our phosphate rock reserves in ways that are more sustainable, equitable and safe for human and environmental health.
Key issue 2.1
Few nations have phosphate rock reserves
The challenge
Five countries hold around 85% of known phosphate rock reserves, with 70% found in Morocco and Western Sahara alone. Most countries do not have any phosphate rock reserves and are reliant on imports to supply their phosphorus demands to maintain food security. China, Morocco and Western Sahara, the USA and Russia currently produce around 80% of the planet’s phosphate rock supply. Whilst China contains less than 5% of the global phosphate rock reserves, it is the largest producer (and consumer) of phosphate rock in the world, accounting for 52% of global phosphate rock production in 2019. However, with ongoing investments in phosphate rock mining in Morocco it is anticipated that Morocco will become the largest producer in the coming years. A high dependency on imported phosphate rock and/or mineral phosphorus fertiliser can contribute to national food system vulnerability. For example, South Asia is almost completely reliant on phosphorus imports. Similarly, most European countries rely heavily on phosphorus imports for mineral fertiliser and animal feed supplements. China, the USA, Russia, Saudi Arabia, and Morocco are among the few countries that contain large phosphate rock reserves and fertiliser production facilities and hence are less reliant on imported phosphorus.
The solution
Replacing mineral phosphorus fertiliser with recycled phosphorus fertiliser would help to shift reliance away from mined phosphorus sources. Optimising capacity to recycle phosphorus throughout the food value chain in combination with societal change (e.g. diet change) would help to reduce phosphorus demand and losses. Enabling mainstream production of sustainable recycled phosphorus fertilisers containing low concentrations of contaminants is an essential prerequisite to upscaling operational recycling. Currently, the use of recovered phosphorus to produce fertiliser cannot compete financially against phosphate rock. To make a significant increase in the use of recovered phosphorus, the mineral fertiliser industry must make a substantial shift to increase its use of recovered phosphorus as a raw material. For example, a goal for fertiliser products to contain a minimum of 20% recycled phosphorus could set a benchmark that demonstrates green commitment across the industry.
Key issue 2.2
Phosphate rock can contain contaminants harmful to human, animal and environmental health
The challenge
Different phosphate rock ores vary in their composition between phosphates, impurities and contaminants. Phosphate rock contaminants can be transferred into fertiliser products, spread on soils, and end up in food. Heavy metals found in phosphate rock include arsenic, cadmium, chromium, uranium, mercury, lead, iron and copper. Phosphate rock can also contain the radionuclides uranium and thorium. Processing phosphate rock into fertiliser can increase the concentration of potentially toxic elements by 1.5 times compared to the original ore. Cadmium is of particular concern as it can pose a risk to human, animal and environmental health when above threshold levels. Cadmium is efficiently retained in the kidney and liver in the human body, with a very long biological half-life ranging from 10 to 30 years, and is classified as a class 1 carcinogen by the International Agency of Research on Cancer and the World Health Organization. Concentrations of cadmium and other metals in soils vary and depend on natural background levels, but increasingly also on human activities, including mineral fertiliser use. The by-products of phosphate rock processing also include ~200 Mt per year of phosphogypsum, which can contain hazardous contaminants. Concerns have been raised that contaminant leaching from phosphogypsum stockpiles may pose a risk to the environment and the health of local communities.
The solution
Internationally agreed limits should be set for cadmium and other harmful contaminants in mineral and recycled phosphorus fertilisers and food. Existing national cadmium limits require better enforcement. Optimising the use of fertiliser to match plant needs alongside practices to reduce phosphorus losses will support a decrease in inputs, thereby further lowering the application of fertiliser contaminants to soils, complementing the use of clean mineral and recycled phosphorus fertilisers.
Key issue 2.3
Geopolitics can impact phosphorus supply and demand, while slowing action on phosphorus sustainability
The challenge
National and regional policies can have direct and indirect impacts on phosphorus access domestically or abroad. This includes taxes, tariffs, trade agreements and legislation. Export restrictions are often subject to extensive public attention and heated debate as they can impact food security. For example, in April 2008, China introduced an export tax of 100-135% on fertilisers to ensure that fertilisers produced in China were used domestically. Political instability in countries mining PR can affect phosphate supply (e.g. Syria). Concerns over the legality/legitimacy of phosphate rock production in Western Sahara remain unresolved. Such issues also contribute to sensitivities (i.e. phosphorus hypersensitivity) that represent a barrier to effective dialogue and action on phosphorus.
The solution
Ensuring phosphate security which would allow all farmers to access sufficient phosphorus to grow crops is a global responsibility and requires international cooperation. Balanced stakeholder participation in negotiations is necessary to ensure phosphate security and avoid domination of regulatory agencies by industries or private interests. In light of the unequal distribution of phosphate rock reserves and the growing need for all states to have access to phosphorus, it would be sensible for states to explore models of governance driven by a benefit-sharing approach, following examples adopted for other natural resources. The approach of addressing common but differentiated responsibilities as used in climate change negotiations could provide a suitable model. An internationally agreed framework promoting sustainable phosphate rock mining and trading is currently missing and urgently needed.
Key issue 2.4
Phosphate rock price spikes remain an ongoing risk
The challenge
In 2008, phosphate rock prices spiked by 800%, causing a subsequent increase in fertiliser prices that affected the livelihood of many of the world’s poorest farmers. This price spike occurred in response to a combination of factors, including instability in energy prices, changing dynamics of supply/demand for agricultural and phosphorus products, and the influence of geopolitics on exports. The stability of phosphate rock prices remains vulnerable to such drivers.
The solution
Stakeholders need to plan for uncertainty by increasing adaptive capacity. Ultimately, improving nutrient management to ensure the most efficient use of phosphorus in agriculture, and improving circularity in national phosphorus cycles will provide the greatest protection against phosphorus supply risk. However, for most nations ‘phosphate independence’ is unrealistic, even impossible; governments, therefore, need to recognise phosphorus supply risks through appropriate policy and regulation.
Key issue 2.5
There is a lack of transparent, complete and comparable phosphate rock data
The challenge
Significant discrepancies in phosphate rock data are reported, making it difficult to assess accurately the risk of geographic depletion of reserves. Differing definitions for phosphate rock ‘reserve’ and ‘resource’ are a cause of discrepancies. Datasets on phosphate rock reserves and resources are commercially sensitive and are often not publicly available. Reserve estimates are dynamic and require regular updating, while conformity in data and reporting is needed. The United States Geological Survey estimates global phosphate rock reserves in 2020 at 70,000 Mt, indicating a current lifetime of >300 years, although a longer lifetime can be expected in practice due to innovation and price elasticity.
The solution
There is a need for transparency and free access to accurate, current data on global reserves and resources of phosphate rock. An independent, international body is needed to assess data regularly and to disseminate findings through appropriate mechanisms, institutions, and outreach programmes. This could serve a similar function for phosphorus supply and demand as the United Nations World Water Quality Alliance does for water quality, by providing governments and other stakeholders with relevant evidence-based assessment, scenarios, solutions, and services. In the meantime, policymakers should be made aware of the discrepancies and uncertainties in phosphate rock data when making policy decisions.
Conclusion
Five countries hold 85% of the planet’s phosphate rock reserves, with 70% found in Morocco and Western Sahara alone. High dependency on imported phosphate rock and/or mineral phosphorus fertiliser can contribute to national food system vulnerability. Whilst most now acknowledge that geological depletion of phosphate rock is not an immediate threat, factors that may impact phosphorus access go beyond physical reserves and include geopolitical, institutional, economic, and managerial factors. An internationally agreed framework promoting sustainable phosphate rock mining and trading is needed. Further to this an independent, international body to assess phosphate rock data and disseminate findings through appropriate mechanisms, institutions and outreach programmes will improve transparency. Improving the efficient use of phosphorus in agriculture and shifting reliance away from mined phosphorus sources by increasing phosphorus recycling may offer the greatest protection against potential phosphorus supply risks. Implementing safe limits for cadmium and other potentially harmful contaminants in all phosphorus fertilisers and feed supplements should be applied globally, especially when considering the global trade in agricultural produce. A goal for fertiliser products to contain a minimum of 20% recycled phosphorus could set a benchmark that demonstrates green commitment across the industry.
Want to read more?
The full chapter contains references to the evidence provided above and acknowledgements of images.
Suggested citation for this chapter: W.J. Brownlie, M.A. Sutton, M.A. de Boer, L. Camprubi, H.A. Hamilton, K.V. Heal, T. Morgandi, T.S. Neset, B.M. Spears. (2021). Chapter 2. Phosphate rock: resources, reserves and uses, in: W.J. Brownlie, M.A. Sutton, K.V. Heal, D.S. Reay, B.M. Spears (eds.), Our Phosphorus Future. UK Centre for Ecology and Hydrology, Edinburgh. doi: 10.13140/RG.2.2.25016.83209